
Palladates – oxide materials based on the element palladium – could be used to make superconductors that work at higher temperatures than cuprates (copper oxides) or nickelates (nickel oxides), according to calculations by researchers at the University of Hyogo, Japan, TU Wien and colleagues. The new study further identifies two such palladates as being “virtually optimal” in terms of two properties important for high-temperature superconductors: the correlation strength and the spatial fluctuations of the electrons in the material.
Superconductors are materials that conduct electricity without resistance when cooled to below a certain transition temperature, Tc. The first superconductor to be discovered was solid mercury in 1911, but its transition temperature is only a few degrees above absolute zero, meaning that expensive liquid helium coolant is required to keep it in the superconducting phase. Several other “conventional” superconductors, as they are known, were discovered shortly afterwards, but all have similarly low values of Tc.
Beginning in the late 1980s, however, a new class of “high-temperature” superconductors with Tc above the boiling point of liquid nitrogen (77 K) emerged. These “unconventional” superconductors are not metals but insulators containing copper oxides (cuprates), and their existence suggests that superconductivity may persist at even higher temperatures. Recently, researchers have identified materials based on nickel oxides as being good high-temperature superconductors in the same vein as their cuprate cousins.
A major goal of this research is to find materials that remain superconducting even at room temperatures. Such materials would greatly improve the efficiency of electrical generators and transmission lines, while also making common applications of superconductivity (including superconducting magnets in particle accelerators and medical devices like MRI scanners) simpler and cheaper.
A fundamental unsolved problem
The classical theory of superconductivity (known as the BCS theory after the initials of its discoverers, Bardeen, Cooper and Schrieffer) explains why mercury and most metallic elements superconduct below their Tc: their fermionic electrons pair up to create bosons called Cooper pairs. These bosons form a phase-coherent condensate that can flow through the material as a supercurrent that does not experience scattering, and superconductivity appears as a result. The theory falls short, however, when it comes to explaining the mechanisms behind high-temperature superconductors. Indeed, unconventional superconductivity is a fundamental unsolved problem in condensed-matter physics.
To better understand these materials, researchers need to know how the electrons of these 3d-transition metals are correlated and how strongly they interact with each other. Spatial fluctuation effects (which are enhanced by the fact that these oxides are typically made as two-dimensional or thin-film materials) are also important. While techniques such as Feynman diagrammatic perturbations can be used to describe such fluctuations, they fall short when it comes to capturing correlation effects like the metal-insulator (Mott) transition, which is one of the cornerstones of high-temperature superconductivity.
This is where a model known as dynamic mean field theory (DMFT) comes into its own. In the new work, researchers led by TU Wien solid-state physicist Karsten Held used so-called diagrammatic extensions to DMFT to study the superconducting behaviour of several palladate compounds.
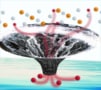
Cuprate superconductors contain a strange component
The calculations, which are detailed in Physical Review Letters, reveal that the interaction between electrons must be strong, but not too strong, to achieve high transition temperatures. Neither cuprates or nickelates are close to this optimum, medium-type interaction, but palladates are. “Palladium is directly one line below nickel in the periodic table,” Held observes. “The properties are similar, but the electrons there are on average somewhat further away from the atomic nucleus and each other, so the electronic interaction is weaker.”
The researchers found that while some palladates, notably RbSr2PdO3 and A′2PdO2Cl2 (A′=Ba0.5La0.5), are “virtually optimal”, others, such as NdPdO2, are too weakly correlated. “Our theoretical description of superconductivity has reached a new level,” Motoharu Kitatani of the University of Hyogo tells Physics World. “We are positive that our experimental colleagues will now try to synthesize these materials.”