Gary Green has spent his career working at the interface between physics, neuroscience and medicine. He speaks to Margaret Harris about starting a company, York Instruments, to commercialize a new brain-imaging technology

How did you get into this field?
I did my PhD in neuroscience at the University of Oxford, UK, having first done an undergraduate degree in bioscience. But when my supervisor offered me the PhD position, he said, “Okay, you’ve done a degree in stamp collecting; now you have to do proper science” – a reference to Ernest Rutherford’s famous comment that all science is either physics or stamp collecting. He had me attend all the undergraduate lectures and problem classes in physics as part of my PhD work in neuroscience. After qualifying in medicine, I worked in medical schools for three decades before I was invited to come to the University of York to set up a brain-imaging centre.
Why did you decide to start a company?
The idea began when the company that made our magnetoencephalography (MEG) machine in the research centre at York went bankrupt, caught out by the collapse of Lehman Brothers in 2008. We had to learn how to maintain it ourselves, and that meant replacing all the electronics because the chips in it were 25 years old – we couldn’t get replacements. That brought us to the attention of a group of Americans who were interested in mild traumatic brain injuries (MTBI), the kind people get from car accidents or playing contact sports such as rugby and American football. They heard that we were building our own MEG electronics, so they approached us and said, “Would you like to join us in forming a company?”
This was in 2014, and I initially refused because I enjoy running the York Neuroimaging Centre and I am still very active in research. But then I thought, “Well, maybe I should try something else in the last part of my career.” Like most academics, I’d done a lifetime of publishing papers and getting grants, but normally, you publish your work and you hope someone else will pick it up and use it. You don’t usually get the opportunity to turn it into something that will help someone.
What are the advantages of MEG over other brain-imaging techniques?
Magnetic resonance imaging (MRI) is great for imaging the brain’s structure, but when people use it to study brain function, they do it indirectly, by looking at changes in the magnetic properties of blood. As soon as an area of the brain becomes active, you get an increase in the flow of oxygenated blood to that area, and when the oxygenation of the haemoglobin in your blood changes, so do its magnetic properties, which affects the MRI signal. But it’s a slow process, one that happens over a period of seconds, and the connection with brain activity is very indirect. It’s a proxy measurement.
One alternative is to stick electrodes on someone’s head and measure the electrical potentials – an electroencephalogram, or EEG. The problem is that when current flows in the brain and creates those differences in electrical potentials, it also flows in layers of tissue over the brain that are not very good at conducting electricity. Although you can sample that electrical activity thousands of times a second, the picture you get is spatially blurred, and it’s also not good at imaging processes that are localized deeper in the brain.
The way to get around this is to image the magnetic fields directly. Wherever there’s current flow, there’s a magnetic field around it. If you have sensors that pick up that magnetic field, you can work out its distribution and solve the inverse problem of where current is flowing. The difficulty is getting sensors that can detect very small magnetic fields, down to femtoteslas (10–15 T). When I was an academic, I met a group of researchers from Royal Holloway, University of London, who had designed a new kind of quantum interference device that could do the job, and we decided to bring this new sensor technology to market in a cheaper, better MEG machine.
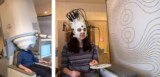
MEG in motion: a wearable brain scanner
What are some of the applications of this technology?
One of the major ones relates to MTBI. Mostly, people with concussion and other MTBI symptoms go to the emergency room and are then sent home, but 40% go on to have long-lasting cognitive effects. That creates two problems. One is that no-one knows who’s going to get these long-lasting effects. The other is that there hasn’t been a good way of detecting what actually happened in the brain to cause them. Almost immediately after a head injury, the brain starts to experience very slow oscillations of electrical current, and therefore of magnetic field. These slow waves last about a second, and conventional imaging machines are not very good recording changes in the magnetic field on this time scale because the technology is too noisy. But our sensors can do it, so that’s very encouraging.
Another potential application is in treatments for epilepsy. Around a third of people with epilepsy are not helped by drugs, and the only alternative for them is surgical – open up the skull, put an array of electrodes over the surface of the brain, find out where the epileptic activity is centred and then remove that area of the brain. It’s highly invasive and high-risk. But if you can localize the epileptic activity with our MEG technique, you can use that information to guide the neurosurgeon, or even use gamma radiation or proton-beam therapy to target that area non-invasively.
What are you working on now?
Scaling up our manufacturing has been a challenge. When we saw these sensors working in the lab, we could see that they were much more sensitive and lower noise than conventional superconducting quantum interference devices (SQUIDs) by a long way, so we thought, “This is fantastic!” But our MEG machine has 350 channels and it’s not practical to make 350 sensors by hand, especially if you want to sell more than one unit. We now make the devices at the wafer scale, with high yields, but it’s taken us two years to get here. We thought it would take a few weeks.
The other thing I’ll mention is that we don’t want to be a one-trick pony, so we are working hard on what our next products will be. We’ve had grants from Innovate UK [a government funding body] to work on optical magnetometers that would replace our novel SQUIDs and allow us to remove the cryogenic system needed to cool them to superconducting temperatures. We’re also developing various software products to help analyse the imaging data.
Any advice for someone thinking of starting a company?
Don’t think you can do it all by yourself. There’s nothing wrong with getting professional business people in to help, and I’ve learned that, as an academic, having a good idea doesn’t necessarily mean you have a good product. Second, you’ve really got to keep up to date with technology. For example, we could have spent five or six thousand dollars on a commercial device to measure magnetic fields in a single channel, meaning that a machine with 350 channels would have been inordinately expensive. By keeping up to date, reading Physical Review Letters or Physical Review, we saw that there was this new device that could measure femtotesla fields, and that you could make it at wafer scale for a few tens of dollars. That’s what allowed us to turn our idea into a commercial device.
- Gary Green is the chief technology officer at York Instruments, e-mail gary.green@york-instruments.com